Structure−function analysis of a phylum-wide conserved glycosyltransferase central to a novel protein O−glycosylation pathway
JOINT-SUPERVISORS: Christina SCHÄFFER, Friedrich ALTMANN
Background.
Protein glycosylation in bacterial systems has come under enhanced scrutiny for two major reasons, i) proven impact of endogenous glycoconjugates on bacterial pathogenicity, with concomitant identification of carbohydrates as lead structures for drug development and ii) establishment of bacteria as easily tractable factories for recombinant glycoprotein production and engineering.
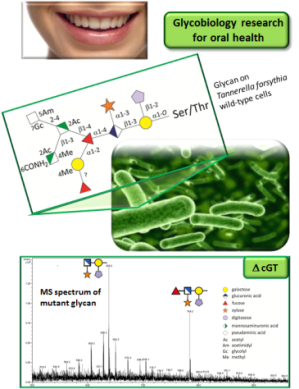
Most
bacterial glycosylation systems rely on a dedicated
oligosaccharyltransferase that ligates the completed glycan chain to
distinct (N− or O−)glycosylation sites of target proteins (Hug et al., 2011). In contrast, for the phylum Bacteroidetes
we have evidence for a so far novel two−step process where a rather
conserved core glycan and a species-specific outer glycan are built up
separately on undecaprenyl-phosphate lipid carriers prior to flipping
across the cytoplasmic membrane and final assembly of the glycan in the
periplasm, followed by eventual transfer onto the novel, Bacteroidetes-specific
three−amino−acid glycosylation motif D(S,T)(A,L,V,I,M,T) by an
O−antigen ligase−like protein (Coyne et al., 2013). This model is
supported at the genome level, where the information for the outer
glycan is encoded by a distinct locus for glycosylation (lfg),
while the information for the core seems to be encoded elsewhere on the
bacterial genome. The organization of genes within the lfgs is
similar, including a highly conserved glycosyltransferase (named cGT)
which is the focus of this study. In successful cross−complementation
experiments targeted at the respective cGT of Tannerella forsythia (periodontal pathogen; cGT encoded by the gene BFO1042), Bacteroides fragilis (intestinal symbiont; cGT encoded by BF4306) and Pedobacter heparinus
(soil bacterium; cGT encoded by PHEP4048), we showed that these cGTs
are functionally interchangeable completely restoring the wild-type
glycosylation patterns of the hypo−glycosylated mutants. According to
preliminary biochemical evidence the cGT would be active at the
intersection between the two converging pathways of core and outer
glycan biosynthesis. Thus, while during evolution many different Bacteroidetes
strains adapted to specific niches as evidenced also by different
protein glycans, the cGT has been obviously maintained, underlining its
central role in the general protein O−glycosylation system.
Aims and methods.
To test our hypothesis of a central GT involved in a novel bacterial protein O−glycosylation system, a structure−function analysis of the enzyme shall be performed. Based on the knowledge of the glycoprotein O−glycan structure in T. forsythia (Posch et al., 2011), the respective BFO1042 cGT shall serve as a basis and will be compared to the B. fragilis system (BF4306 cGT) for which O−glycan information is still limited (Coyne et al., 2013). BFO1042 codes for a 255 aa protein that is classified as a GT family 2 protein according to CAZy and predicted to have a (α/β)8-barrel fold. It possesses three potential metal binding sites and displays one Bacteroidetes O−glycosylation motif. These features are conserved in the B. fragilis cGT. BFO1042 (T. forsythia) and BF4306 (B. fragilis) share 58% amino acid identity.
Experimental set-up:
(i) Determination of potential nucleotide sugar substrates according to the O−glycan structures of T. forsythia and B. fragilis
as well as their endogenous nucleotide sugar pools (Pabst et al.,
2010). This is based on MS structure analysis of the truncated O−glycan
produced in the T. forsythia ΔBFO1042 mutant as well as refinement of the B. fragilis
wild−type O−glycan structure and analysis of the corresponding ΔBF4306
mutant structure. MS−methods will be adapted to meet the requirements
for analysis of glycans and sugar precursors with low abundance and
unusual, bacterial, constituents.
(ii) Optimization of recombinant cGT production in E. coli
with a cleavable tag for purification. Considering the presence of
potential glycosylation sites, the cGTs will also be produced in tagged
form in the homologous systems (Posch et al., 2013); in case
glycosylation is detected, these enzymes will be compared biochemically
to their non−glycosylated counterparts.
(iii) Structure−function analysis of the enzyme. To gain insight into
the biochemistry and structure of the cGT, spectral (e.g. ECD)
investigations will be performed. Once potential nucleotide sugar
substrates for the cGT have been identified (see (i)), and, if
necessary, isolated from bacterial cultures, an in vitro enzyme
assay will be set up, using purified glycopeptides produced by the
mutants as acceptors. Data will be complemented by enzyme steady-state
kinetic studies.
(iv) Given the predicted novelty of the cGTs, but depending on the
progress of the work, protein modelling and nucleotide sugar docking
studies are intended.
(vi) To unravel a potential physiological relevance of the conserved
core glycan present on various proteins of the ΔcGT mutants, the T. forsythia
ΔBFO1042 mutant will be analyzed in comparison to the wild−type for its
capacity to form biofilm as well as to invade and adhere to oral
epithelial cells.
Summing up, this project will deliver information about a key enzyme of an obviously novel general protein O−glycosylation
system elaborated by microbes with various physiologies.
Simultaneously it can pinpoint a novel target for interfering with
glycan-mediated phenomena relevant to these microbes, such as in the
pathogenesis of periodontitis.
Collaborations within this thesis will include
OBINGER (biospectroscopy) and OOSTENBRINK (sugar nucleotide docking
studies and protein modelling). International cooperations include
BELIBASAKIS (multispecies biofilm studies) and STAFFORD (cell
invasion/adhesion assays).
Coyne,
M. J., Fletcher, C. M., Chatzidaki-Livanis, M., Posch, G., Schäffer,
C., Comstock, L. E. (2013) Phylum−wide general protein O−glycosylation system of the Bacteroidetes. Mol. Microbiol. 88, 772-783.
Hug, I. and Feldman, M. F. (2011) Analogies and homologies in
lipopolysaccharide and glycoprotein biosynthesis in bacteria.
Glycobiology 21, 138-151.
Pabst, M., Grass, J., Fischl, R., Leonard, R., Jin, C., Hinterkorner,
G., Borth, N., Altmann, F. (2010) Nucleotide and nucleotide sugar
analysis by liquid chromatography−electrospray ionization−mass
spectrometry on surface−conditioned porous graphitic carbon. Anal. Chem.
82, 9782-9788.
Posch, G., Pabst, M., Brecker, L., Altmann, F., Messner, P., Schäffer, C. (2011) Characterization and scope of S−layer protein O-glycosylation in Tannerella forsythia. J. Biol. Chem. 286, 38714-38724.
Posch, G., Pabst, M., Neumann, L., Coyne, M. J., Altmann, F., Messner,
P., Comstock, L. E., Schäffer, C. (2013) Cross−glycosylation of proteins
in Bacteroidales species. Glycobiology 23, 568-577.