Understanding the source of variation in protein N-glycosylation patterns of recombinant proteins generated in CHO cells
JOINT-SUPERVISORS: Nicole BORTH, Richard STRASSER
Background.
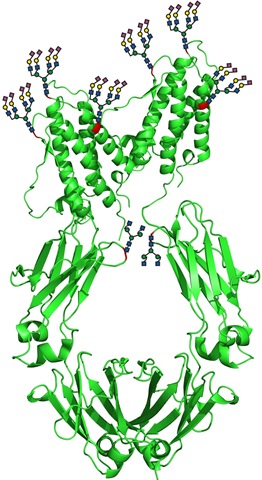
A very important quality parameter for recombinant therapeutic proteins produced in mammalian cell lines is glycosylation (Hayes et al., 2014). It has direct impacts on protein conformation and structure, thus influencing biological activity and the presence or absence of certain sugar residues may cause unwanted immune responses (Byrne et al., 2007). Specific glycosylation patterns vary between animal species, but also between individual production clones (e.g. due to clonal differences in gene expression in CHO cells) and even between production process runs, due to changes in environmental conditions and the ensuing response in the transcriptome (Aghamohseni et al., 2014). Thus selecting the right clone and optimising the process conditions to maintain consistent product quality is a major task of the biopharmaceutical industry to ensure safe and efficient therapies. This is a major challenge, as different proteins require different types of glycosylation and even on the same protein different glycosylation sites may have specific glycans attached that are unique for each site (Taschwer et al., 2012) .
The source of the variation is currently not well understood, neither on the level of clonal variation, nor on the level of environmental response nor on the level of protein- and site-specific glycosylation. Several possible sources of this variation are known including difference in terms of genome, epigenome and translatome so that the specific expression of glycosylation enzymes may impact on the relative abundance of certain modifications (Lewis et al., 2013; Pilbrough et al., 2009). In addition, the overall complexity of glycosylation pathways with competition between glycosyltransferases contributes to variation. However, experience with mutants deficient in distinct glycosylation steps show that uniform glycosylation patterns can be obtained in CHO cells. Recent analysis of the CHO genome and a global view on glycosylation gene expression under experimental growth conditions allow rationally designed strategies to optimize and control glycosylation in CHO cells (Lewis et al., 2013; Xu et al., 2011).
Aims and methods.
The main objective of this project is to investigate the contribution of distinct N-glycan processing enzymes in the Golgi to the production of homogeneous glycan structures. The knowledge generated aims at increasing our understanding of glycosylation processes in CHO cells for reliable production of high-quality pharmaceuticals with optimized N-glycosylation. CRISPR-based genome editing tools and gene overexpression will be used to generate two CHO cell lines with defined glycosylation patterns:
a) Generation of CHO cells for production of glycoproteins with human-type sialylation: a minimal genome editing strategy will be designed to simultaneously (i) inactivate CMP-Neu5Ac hydroxylase - to prevent the incorporation of the immunoreactive N-glycolylneuraminic acid (Neu5Gc), (ii) eliminate genes coding for secreted sialidases to prevent extracellular cleavage of terminal sialic acid and (iii) mutate UDP-GlcNAc 2-epimerase (GNE) to prevent feedback-regulation of CMP-Neu5Ac biosynthesis. In addition, human α2,6-sialyltransferase will be overexpressed or the endogenous C. griseus enzyme activated to generate N-glycans with human-type α2,6-linked sialic acid.
b) Generation of CHO cells for production of glycoproteins with homogeneous tetra-antennary N-glycans: To increase the sialic acid content expression of the underrepresented endogenous C. griseus N-acetylglucosaminyltransferase IV will be increased or the corresponding human enzyme will be overexpressed in CHO cells engineered for human-type sialylation.
Two model proteins will be transiently produced in the engineered cell lines: Epo-Fc (Taschwer et al., 2012), which has two N-glycosylation sites and one O-glycosylation site on the EPO part of the protein where these N-glycans are highly complex consisting of 20% bi-antennary, 40% tri-antennary and 40% tetra-antennary structures. The Fc-part of the protein has one N-glycosylation site consisting exclusively of bi-antennary, non-sialylated glycans. The second model protein is human diaminooxidase which bears 4 N-glycosylation sites of which 3 are consistently complex-type while one is high mannose. Proteins will be purified and glycan structures of the two model proteins produced in the generated CHO cell lines will be analysed by LC-ESI-MS and compared to proteins produced in wild-type cells as well as to total N-glycans of endogenous proteins from cell extracts. Functional assays like receptor binding (Epo-Fc) and enzyme activity assays (diaminooxidase) will be carried out to test the influence of the glycans on protein function. Growth and productivity of the engineered cell lines will be compared, transcript expression of glycosylation enzymes will be quantified and the influence of culture conditions on glycan homogeneity and the generation of defined N-glycan structures will be investigated to identify potential targets for further improvement. The following outcomes are expected for the project: a) generation of CHO cell variants with defined glycosylation patterns; and b) a detailed understanding of the impact of individual glycosylation enzymes on the glycosylation pattern of recombinant and endogenous proteins, in the context of specific protein structures. The obtained information can be further used to improve currently existing genome scale models for CHO by integrating information on glycosylation pathways.
This project will be headed by BORTH (CHO cell engineering, CRISPR) and will be performed in close collaboration with STRASSER (eukaryotic glycosylation pathways, glycoengineering). Further collaborations within this thesis will include ALTMANN (mass spectrometry and glycan analysis), ZANGHELLINI (elementary flux mode analysis) and OOSTENBRINK (modelling of proteins and glycans). Possible laboratories for external stay: MIKE BETENBAUGH, Johns Hopkins University, USA, (eukaryotic glycosylation); HELENE FAUSTRUP KILDEGAARD, DTU, Denmark (CRISPR technology); NATHAN LEWIS, UCSD, USA (genome scale models) or DONG-YUP LEE, University of Singapore and a*-star (CHO cell engineering).
Hayes JM et al. (2014) Glycosylation and Fc-receptors. Curr Top Microbiol Immunol 382, 165-199
Byrne B et al. (2007) Sialic acids: carbohydrate moieties that influence the biological and physical properties of biopharmaceutical proteins and living cells. Drug Discovery today 12:7-8, 319-326
Aghamohseni H et al. (2014) effects of nutrient levels and average culture pH on the glycosylation pattern of camelid-humanized monoclonal antibody. J Biotechnol 186, 98-109
Taschwer M et al.(2012) Growth, productivity and protein glycosylation in a CHO EpoFc producer cell line adapted to glutamine- free growth. J Biotechnol. 157, 295-303
Lewis NE et al. (2013) Genomic landscapes of Chinese hamster ovary cell lines as revealed by the Cricetulus griseus draft genome. Nat. Biotechnol. 31, 759–765
Pilbrough W et al . (2009) Intraclonal protein expression heterogeneity in recombinant CHO cells. PLoS One, 4, e8432
Baycin-Hizal D et al. (2014) Physiologic and pathophysiologic consequences of altered sialylation and glycosylation on ion channel function. Biochem Biophys Res Commun 453, 243-253
Xu X et al. (2011) The genomic sequence of the Chinese hamster ovary (CHO)-K1 cell line. Nat. Biotechnol. 29, 735-741