Rational systems design of protein-producing yeasts: eliminating metabolic limitations
JOINT-SUPERVISORS: Brigitte GASSER, JÜRGEN ZANGHELLINI, Dietmar HALTRICH, Diethard MATTANOVICH
Background.
The production of recombinant proteins is frequently enhanced at the levels of transcription, codon usage, protein folding and secretion (Delic et al., 2014). Primary metabolism, on the other hand, is hardly targeted despite experimental evidence that it limits recombinant protein production (e.g. Heyland et al., 2011 for Pichia pastoris). One reason for neglecting the central metabolism is its inherent flexibility, which requires extensive systems level insight − typically gained through (genome-scale) computational modelling − to identify appropriate engineering targets. After closing this gap by developing the first genome−scale metabolic model of P. pastoris (Sohn et al., 2010), we used this computational model together with constraint-based analysis to rationally predict single cell engineering targets that channel available resources towards protein production. In BioToP’s first funding period, these predictions were experimentally verified (Nocon et al., 2014). Thus for the first time systems biotechnological analyses and rational design processes based on genome-scale metabolic modelling were successfully utilized to improve recombinant protein production in P. pastoris. Interestingly, many of the predicted genetic modifications were situated in the pentose phosphate pathway (PPP) and found to strongly modify the redox and energy metabolism. In particular, a higher pool of reduced NADPH positively correlated to improved recombinant protein production. Currently, fluxomics analysis of these improved strains is performed to better understand the complex interplay and the trade−off between redox and energy balance (Nocon et al., in preparation).
Aims and methods.
This interdisciplinary systems biology project aims at rationally predicting engineering targets that alter the cofactor balance in order to better meet the demands of recombinant protein production, engineering the appropriate enzymes, and implementation as well as experimental characterisation of the resulting production strains. To reach this aim the project combines computational modelling and targeted engineering with omics analysis.
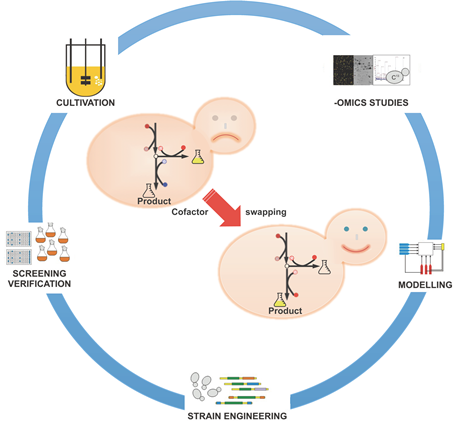
Based on our findings that the cofactor balance (NAD/H to NADP/H) in P. pastoris does not match the needs for optimized recombinant protein production, this project aims to find additional cellular targets that alter this balance towards higher NADPH pools. As the number of NADP-reducing enzymes in the cells is limited (mainly PPP enzymes), we propose to change cofactor specificity of selected oxidoreductases from NAD/H to NADP/H. Cofactor switching between NAD/H and NADP/H was successfully realised in vivo in different yeast and bacterial strains (Hasegawa et al., 2012; Petschacher and Nidetzky, 2008; Martinez et al., 2008; Verho et al., 2003), proofing the feasibility of this strategy, but has not yet been performed for P. pastoris or in the context of improved recombinant protein production. In order to reduce wet lab efforts, metabolic modelling will be employed to predict the most promising oxidoreductases (1-3 candidates) for such purposes.
To this end the recently generated fluxomics data of the improved strains will be integrated into the available genome-scale metabolic model of P. pastoris together with constraint-based analysis (Gerstl et al.,2015) to model their phenotypes (Lewis et al., 2012). The such updated genome-scale metabolic model of P. pastoris will then be analysed with the tool “OptSwap” (King and Feist, 2013). OptSwap allows one to identify global, optimal cofactor-specificity swaps for increasing the theoretical product yield in genome-scale metabolic models. From a theoretical point, the reactions catalyzed by suitable candidate oxidoreductases should have a high flux, and preferably protein structures of orthologs from other species should be available. Rational candidates could include isocitrate dehydrogenase, glycerol 3-phosphate dehydrogenase or glyceraldehyde 3-phosphate dehydrogenase, for all of which both NAD/H as well as NADP/H dependent isoforms are known to exist in different organisms. Metabolic modelling will be employed to test whether these are indeed suitable candidates or whether other, better candidate oxidoreductases can be identified. Based on these bioinformatics predictions, 1-3 of the most promising enzymes will be replaced in vivo with an engineered and optimized non-native oxidoreductase with specificity for the NADP/H redox cofactor. As backup, NADP/H-dependent isoforms from other species could be employed (e.g. Martinez et al., 2008). The resulting strains will be experimentally characterized and their production properties verified. Finally, the result of this omics analysis should be re-fed into the computational modelling pipeline for further analysis. We expect that the results will give important first indications as to whether altering the coenzyme balance is an appropriate and promising approach for improving recombinant protein production in P. pastoris.
Collaborations: Computational modelling will take place in the group of Jürgen ZANGHELLINI. Enzyme engineering will take place in the group of Dietmar HALTRICH. Experimental strain modification and strain characterization including metabolomics and fluxomics data interpretation will be performed in the groups of Brigitte GASSER and Diethard MATTANOVICH. Metabolome and fluxome will be measured in the group of Gunda KÖLLENSPERGER (for methods see: Klavins et al., 2013; Guerrasio et al., 2013). A stay abroad at the Autonomous University of Barcelona (Spain) in Pau FERRER's lab (e.g. Baumann et al., 2010) is anticipated (6 months).
Baumann, K., Carnicer, M., Dragosits, M., Graf, A. B., Stadlmann, J., Jouhten, P., Maaheimo, H., Gasser, B., Albiol, J., Mattanovich, D., Ferrer. (2010) A multi-level study of recombinant Pichia pastoris in different oxygen conditions. BMC Syst. Biol. 4,141
Delic, M., Göngrich, R., Mattanovich, D., Gasser B. (2014) Engineering of protein folding and secretion-strategies to overcome bottlenecks for efficient production of recombinant proteins. Antioxid. Redox Signal. 21,414-37
Gerstl, M. P., Ruckerbauer, D. E., Mattanovich, D., Jungreuthmayer, C., Zanghellini, J. (2015) Metabolomics integrated elementary flux mode analysis in large metabolic networks. Sci. Rep. 5:8930
Guerrasio, R., Haberhauer-Troyer, C., Steiger, M., Sauer, M., Mattanovich, D., Koellensperger, G., Hann, S. (2013) Measurement uncertainty of isotopologue fractions in fluxomics determined via mass spectrometry. Anal. Bioanal. Chem. 405,5133-5146
Hasegawa, S., Uematsu, K., Natsuma, Y., Suda, M., Hiraga, K., Jojima, T., Inui, M., Yukawa, H. (2012) Improvement of the redox balance increases L-valine production by Corynebacterium glutamicum under oxygen deprivation conditions. Appl. Environ. Microbiol. 78,865-75
Heyland, J., Blank, L. M., Schmid, A. (2011) Quantification of metabolic limitations during recombinant protein production in Escherichia coli. J. Biotechnol. 155:178-84
Lewis, N. E., Nagarajan, H., Palsson, B. O. (2012) Constraining the metabolic genotype–phenotype relationship using a phylogeny of in silico methods. Nat. Rev. Microbiol. 10,291-305
Klavins, K., Neubauer, S., Al Chalabi, A., Sonntag, D., Haberhauer-Troyer, C., Russmayer, H., Sauer, M., Mattanovich, D., Hann, S., Koellensperger, G. (2013) Interlaboratory comparison for quantitative primary metabolite profiling in Pichia pastoris metabolomics and Metabolite Profiling. Anal. Bioanal. Chem. 405,5159-5169
King, Z. A., Feist, A. M. (2013) Optimizing Cofactor Specificity of Oxidoreductase Enzymes for the Generation of Microbial Production Strains—OptSwap. Industrial Biotechnology 9: 236-246
Martinez, I., Zhu, J., Lin, H., Bennett, G. N., San, K. Y. (2008) Replacing Escherichia coli NAD-dependent glyceraldehyde 3-phosphate dehydrogenase (GAPDH) with a NADP-dependent enzyme from Clostridium acetobutylicum facilitates NADPH dependent pathways. Metab. Eng. 10,352–359
Nocon, J., Steiger, M. G., Pfeffer, M., Sohn S. B., Kim, T. Y., Maurer, M., Russmayer, H., Pflügl, S., Haberhauer-Troyer, C., Ortmayr, K., Hann, S., Koellensperger, G., Gasser, B., Lee, S. Y., Mattanovich, D. (2014) Model based engineering of Pichia pastoris' central metabolism enhances recombinant protein production. Metab. Eng. 24,129-138
Petschacher, B., Nidetzky, B. (2008) Altering the coenzyme preference of xylose reductase to favor utilization of NADH enhances ethanol yield from xylose in a metabolically engineered strain of Saccharomyces cerevisiae. Microb. Cell Fact. 7:9
Sohn, S. B., Graf, A. B., Kim, T. Y., Gasser, B., Maurer, M., Ferrer, P., Mattanovich, D., Lee, S. Y. (2010) Genome-scale metabolic model of methylotrophic yeast Pichia pastoris and its use for in silico analysis of heterologous protein production. Biotechnol. J. 5,705-15
Verho, R., Londesborough, J., Penttilä, M., Richard, P. (2003) Engineering redox cofactor regeneration for improved pentose fermentation in Saccharomyces cerevisiae. Appl. Environ. Microbiol. 69:5892