Methylated N-glycans in moss – annoying or useful for biotechnology?
SUPERVISOR: Friedrich ALTMANN
Background.
The glycome and glyco-genome, i.e. the array of genes involved in protein glycosylation, of mammals, especially humans, is by now well defined. This knowledge slowly, but inexorably yields insights into the biological role of protein glycosylation (Varki, 2017). As an example, a recent study on the cause of ricin resistance of mutant cell lines shall be mentioned (Stadlmann et al., 2017). Much hope rests on anti-HIV antibodies that are broadly neutralizing because they target glycan epitopes as these are only minimally affected by single mutations (Crispin et al., 2018; Crooks et al., 2018). Vice versa, glycosylation is regarded as a key element in the development of anti-HIV vaccines (Doores, 2015).
More generally, the purpose of vaccination is to elicit a strong immune response towards the vaccine. This is a particular challenge in the case of recombinant protein vaccines for which a variety of adjuvant strategies have been developed to overcome their inherently poor immunogenicity (Moyle, 2017). Pertinent to this application are two strategies: Mixing with or coupling to an immune-stimulatory glycoprotein (Zhong et al., 2016; Anzengruber et al., 2017) or introduction of non-mammalian glyco-features (Sandig et al., 2017).
The glycome of green plants has been firmly established over the last decades. Apart from xylose it is especially the core a1,3-linked fucose that distinguishes plant from human glycans (Gomord et al., 2005; Altmann, 2007). Analysis of a large number of samples from different plant species including angio- and gymnosperms, ferns and horsetails and even a moss tissue culture consistently produced the same familiar pattern (Koprivova et al. 2004). Recently, however, we analysed mosses from the order Hypnales (exact species determination ongoing with Dr. Harald G. Zechmeister from the Department of Botany and Biodiversity Research, University of Vienna), and found that - though otherwise impeccably plant-like – their hybrid-type N-glycans are O-methylated (unpublished results). O-methylated N-glycans have so far only been found in bacteria and “lower animals” such as snails, in plant polysaccharides but not in plant glycoproteins (Staudacher, 2012).
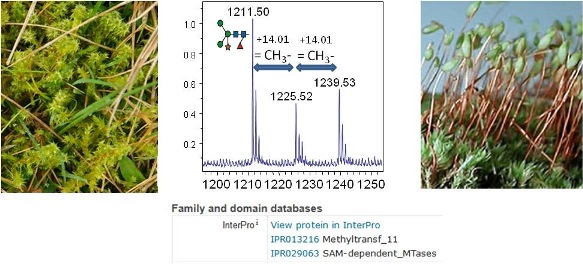
Aims and methods.
In the first part of thesis, the N-glycan structures of Rhytidiadelphus squarrosus and other easily available moss species will be thoroughly elucidated by LC-MS and other relevant methods as previously practiced (Altmann et al., 2016). The immunogenicity of these methylated glycans will be studied in rabbits with the help of neo-glycoproteins containing moss glycopeptides and – for comparison – mammalian glycopeptides trimmed to a similar yet non-methylated structure in a way similar to the study on the immunogenicity of plant N-glycans (Bencurova et al., 2004). At first, this shall reveal if anti-glycan antibodies are generated towards methylated versions of these otherwise immune-inert oligosaccharides. Additionally, we will examine to what extent this non-human feature modulates the immune response to the carrier protein (e.g. HSA).
In the second part of this thesis, the enzyme(s) responsible for this new modification, i.e. the moss methyl-to-mannose transferase shall be identified. This shall be undertaken by two strategies. The first one will be rather biochemical, trying to enrich and measure the activity of the methyl transferase. LC-MS based proteomics shall then identify enriched SAM-cassette-containing proteins. A prerequisite for this work is the availability of sequences. In the absence of annotated genomes of the species investigated we will resort to transcriptome analysis as practized recently for a yeast (Blasi et al. 2017). The work shall finally be completed by recombinant expression (probably in the baculovirus-insect cell system) of a moss methyl transferase and by confirming and characterizing its activity. This will allow the avoidance of unwanted methylation where a moss is used as expression vector or make use of it as an intrinsic adjuvant.
Collaboration within this thesis will include GRABHERR (immunogenicity assessment)
Altmann, F. (2007) The role of protein glycosylation in allergy. Int. Arch. Allergy Immunol. 142, 99-115. doi:10.1159/000096114
Altmann, F., Kosma, P., O'Callaghan, A., Leahy, S., Bottacini, F., Molloy, E., Plattner, S., Schiavi, E., Gleinser, M., Groeger, D., Grant, R., Rodriguez Perez, N., Healy, S., Svehla, E., Windwarder, M., Hofinger, A., O'Connell Motherway, M., Akdis, C. A., Xu, J., Roper, J., van Sinderen, D., O'Mahony, L. (2016) Genome Analysis and Characterisation of the Exopolysaccharide Produced by Bifidobacterium longum subsp. longum 35624." PLoS One 11: e0162983. doi:10.1371/journal.pone.0162983
Anzengruber, J., Bublin, M., Bonisch, E., Janesch, B., Tscheppe, A., Braun, M. L., Varga, E. M., Hafner, C., Breiteneder, H., Schaffer, C. (2017) Lactobacillus buchneri S-layer as carrier for an Ara h 2-derived peptide for peanut allergen-specific immunotherapy. Mol. Immunol. 85, 81-88. doi: 0.1016/j.molimm.2017.02.005
Bencurova, M., Hemmer, W., Focke-Tejkl, M., Wilson, I.B.H., Altmann, F. (2004) Specificity of IgG and IgE antibodies against plant and insect glycoprotein glycans determined with artificial glycoforms of human transferrin. Glycobiology 14, 457-466. doi: 10.1093/glycob/cwh058
Blasi, B., Tafer, H., Kustor, C., Poyntner, C., Lopandic, K., Sterflinger, K. (2017) Genomic and transcriptomic analysis of the toluene degrading black yeast Cladophialophora immunda. Sci. Rep. 7, 11436. doi: 10.1038/s41598-017-11807-8
Crispin, M., Ward, A. B., Wilson, I.A. (2018) Structure and Immune Recognition of the HIV Glycan Shield. Annu. Rev. Biophys. 47, 499-523. doi:10.1146/annurev-biophys-060414-034156
Crooks, E.T., Grimley, S.L., Cully, M., Osawa, K., Dekkers, G., Saunders, K., Rmisch, S., Menis, S., Schief, W.R., Doria-Rose, N., Haynes, B., Murrell, B., Cale, E. M., Pegu, A., Mascola, J.R., Vidarsson, G., Binley, J.M. (2018) Glycoengineering HIV-1 Env creates 'supercharged' and 'hybrid' glycans to increase neutralizing antibody potency, breadth and saturation. PLoS Pathog. 14, e1007024. doi:10.1371/journal.ppat.1007024
Doores, K.J. (2015) The HIV glycan shield as a target for broadly neutralizing antibodies. FEBS J. 282, 4679-4691. doi:10.1111/febs.13530
Gomord, V., Chamberlain, P., Jefferis, R., Faye, L. (2005) Biopharmaceutical production in plants: problems, solutions and opportunities. Trends Biotechnol. 23, 559-565. doi: 10.1016/j.tibtech.2005.09.003
Koprivova, A., Stemmer, C., Altmann, F., Hoffmann, A., Kopriva, S., Gorr, G., Reski, R., Decker, E.L. (2004) Targeted knockouts of Physcomitrella lacking plant-specific immunogenic N-glycans. Plant Biotechnol. J. 2, 517-523. doi:10.1111/j.1467-7652.2004.00100.x
Moyle, P.M. (2017) Biotechnology approaches to produce potent, self-adjuvanting antigen-adjuvant fusion protein subunit vaccines. Biotechnol. Adv. 35, 375-389. doi: 10.1016/j.biotechadv.2017.03.005
Sandig, G., von Horsten, H. H., Radke, L., Blanchard, V., Frohme, M., Giese, C., Sandig, V., Hinderlich, S. (2017) Engineering of CHO Cells for the Production of Recombinant Glycoprotein Vaccines with Xylosylated N-glycans. Bioengineering (Basel) 4, E38. doi: 10.3390/bioengineering4020038
Stadlmann, J., Taubenschmid, J., Wenzel, D., Gattinger, A., Durnberger, G., Dusberger, F., Elling, U., Mach, L., Mechtler, K., Penninger, J.M. (2017) Comparative glycoproteomics of stem cells identifies new players in ricin toxicity. Nature 549, 538-542. doi: 10.1038/nature24015
Staudacher, E. (2012) Methylation - an uncommon modification of glycans. Biol. Chem. 393, 675-685. doi: 10.1515/hsz-2012-0132
Varki, A. (2017) Biological roles of glycans. Glycobiology 27, 3-49. doi: 10.1093/ glycob/cww086
Zhong, T.Y., Arancibia, S., Born, R., Tampe, R., Villar, J., Del Campo, M., Manubens, A., Becker, M.I. (2016) Hemocyanins Stimulate Innate Immunity by Inducing Different Temporal Patterns of Proinflammatory Cytokine Expression in Macrophages. J. Immunol. 196, 4650-4662. doi: 10.4049/jimmunol.1501156