Folding of recombinant bacterial RuBisCO
SUPERVISOR: DIETHARD MATTANOVICH
Background.
The enzyme ribulose-1,5-bisphosphate carboxylase/oxygenase (RuBisCO) accounts for assimilation of almost all inorganic carbon in the form of CO2 in the atmosphere and represents the most abundant protein on earth (Ellis 1979). The fact that RuBisCO conquered its predominant role among the CO2 fixation routes remains puzzling, especially with focus on its major drawbacks: (i) RuBisCO needs to be activated post-translationally to carry out the carboxylation reaction; (ii) it has a low turnover frequency which can limit CO2 fixation; (iii) it is prone to carry out “false” reactions leading to inhibiting side products (Erb and Zarzycki, 2018; Parry et al., 2007; Hazra et al., 2015; Vitlin Gruber and Feiz, 2018).
The role of the form I RuBisCO foldases and activases have been well studied (Salvucci et al., 1985; Mueller-Cajar et al., 2014; Portis & Jr., 2003; recently reviewed by Bracher et al., 2017). Chemoautotrophic proteobacteria, however, often possess several forms of RuBisCO (Badger and Bek, 2008), but lack typical RuBisCO activases or CbbX genes. Instead, these organisms are often equipped with two proposed activases encoded by the open reading frames CbbQ and CbbO, which are in close proximity to the RuBisCO genes (Hayashi et al., 1997; Badger and Bek 2008). The role of these open reading frames in association with form II RuBisCO activity was recently elucidated and was found to have activase activity (Tsai et al., 2015). Interestingly, the heterologous expression of RuBisCO in bacteria and yeast with and without these activases lead to contradicting results either showing a beneficial (Hayashi et al., 1999) or no significant (Guadalupe-Medina et al., 2013) impact on RuBisCO activity.
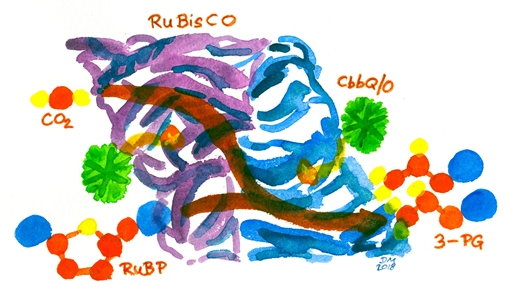
On the contrary, it was shown that the Escherichia coli chaperones GroEL and GroES lead to an activation of form II RuBisCO (Guadalupe-Medina et al. 2013). The role of native GroEL/GroES chaperones on folding of form II RuBisCO, however, was not investigated. These puzzling results encourage us to further investigate the effect of different chaperone systems in an orthogonal yeast system. We hypothesize that GroEL/GroES are necessary for folding of the monomers while CbbQ/CbbO activates RuBisCO downstream of folding and assembly.
Aims and methods.
In this project, we will study the impact of different chaperone systems on folding and activation of a type II RuBisCO from Thiobacillus denitrificans as a model protein. Based on this RuBisCO, we have recently successfully established a fully functional Calvin-Benson-Bassham cycle in the methylotrophic yeast Komagataella phaffii (syn. Pichia pastoris) (unpublished, manuscript in preparation). In contrast to a yeast system expressing RuBisCO in Saccharomyces cerevisiae (Guadalupe-Medina et al., 2013), the engineered K. phaffii can readily grow on CO2 as carbon source. This demonstrates that RuBisCO is correctly folded and active in the heterologous host, so that it is an ideal orthogonal system to study the impact of different chaperone systems on the overall RuBisCO activity.
Three HSP60/10 chaperone systems (the GroEL/GroES homologs of T. denitrificans and E. coli respectively, and HSP60/10 of K. phaffii mitochondria), as well as CbbQ/CbbO of T. denitrificans will be characterized for their capability to fold and activate RuBisCO.
For in vivo testing, the chaperone systems will be expressed in K. phaffii harboring a functional CBB cycle, and the growth behavior in the presence and absence of the chaperones will be assessed. Specific RuBisCO activities as well as in vivo interaction with chaperones and multimerization of RuBisCO will be quantified by native PAGE, Western blotting, and activity assays following Tsai et al. (2015) and Aigner et al. (2017).
For in vitro testing, the chaperones as well as the RuBisCO will be produced in the K. phaffii protein expression platform in collaboration with GASSER (Gasser et al., 2013) and His-tag purified. Additionally, in vitro translation of these proteins will be evaluated. The impact of different combinations of chaperones/activases on overall structure, oligomeric state and (conformational and thermal) stability of RuBisCO will be monitored by CD and fluorescence spectroscopy, SEC-MALS, and DSC (in cooperation with OBINGER).
In collaboration with OOSTENBRINK, a structural model of RuBisCO will be created by comparative modeling against RuBisCO from Gallionella sp. (87% sequence identity). The stability of a single monomer will be studied by performing molecular dynamics simulations under different conditions.
Collaborations within this thesis will include GASSER (expression in K. phaffii) OBINGER (protein structure) and OOSTENBRINK (molecular modeling).
Aigner, H., Wilson, R.H., Bracher, A., Calisse, L., Bhat, J.Y., Hartl, F.U., Hayer-Hartl, M. (2017) Plant RuBisCo assembly in E. coli with five chloroplast chaperones including BSD2. Science 358, 1272-1278. doi: 10.1126/science.aap9221.
Badger, M.R. and Bek, E.J. (2008) Multiple Rubisco forms in proteobacteria: their functional significance in relation to CO2 acquisition by the CBB cycle. J. Exp. Bot. 59, 1525–1541. doi: 10.1093/jxb/erm297
Bracher, A., Whitney, S.M., Hartl, F.U., Hayer-Hartl, M. (2017) Biogenesis and metabolic maintenance of Rubisco. Annu. Rev. Plant. Biol. 68, 29-60. doi: 10.1146/annurev-arplant-043015-111633
Ellis, R.J. (1979) The most abundant protein in the world. Trends Biochem. Sci. 4, 241-244.
Erb, T.J. and Zarzycki, J. (2018) A short history of RubisCO: the rise and fall (?) of Nature’s predominant CO2 fixing enzyme. Curr. Opin. Biotechnol. 49, 100-107. doi: 10.1016/j.copbio.2017.07.017
Gasser, B., Prielhofer, R., Marx, H., Maurer, M., Nocon, J., Steiger, M., Puxbaum, V., Sauer, M., Mattanovich, D. (2013) Pichia pastoris: protein production host and model organism for biomedical research. Future Microbiol. 8, 191-108. doi: 10.2217/ fmb.12.133
Guadalupe-Medina, V., Wisselink, H.W., Luttik, M.A., de Hulster, E., Daran, J.M., Pronk, J.T., van Maris, A.J. (2013) Carbon dioxide fixation by Calvin-Cycle enzymes improves ethanol yield in yeast. Biotechnol. Biofuels 6, 125. doi: 10.1186/1754-6834-6-125
Hayashi, N.R., Arai, H., Kodama, T., Igarashi, Y. (1997) The novel genes, cbbQ and cbbO, located downstream from the RubisCO Genes of Pseudomonas hydrogenothermophila, affect the conformational states and activity of RubisCO. Biochem. Biophys. Res. Commun. 241, 565–569. doi:10.1006/bbrc.1997.7853
Hayashi, N.R., Arai, H., Kodama, T., Igarashi, Y. (1999) The cbbQ genes, located downstream of the form I and form II RubisCO genes, affect the activity of both RubisCOs. Biochem. Biophys. Res. Commun. 265, 177-183. doi:10.1006/bbrc.1999.1103
Hazra, S., Henderson, J.N., Liles, K., Hilton, M.T., Wachter, R.M. (2015) Regulation of ribulose-1,5-bisphosphate carboxylase/oxygenase (rubisco) activase: product inhibition, cooperativity, and magnesium activation. J. Biol. Chem. 290, 24222-24236. doi: 10.1074/jbc.M115.651745
Mueller-Cajar, O., Stotz, M., Bracher, A. (2014) Maintaining photosynthetic CO2 fixation via protein remodelling: the Rubisco activases. Photosynth. Res. 119, 191-201. doi: 10.1007/s11120-013-9819-0
Parry, M.A., Keys, A.J., Madgwick, P.J., Carmo-Silva, A.E., Andralojc, P.J. (2008) Rubisco regulation: a role for inhibitors. J. Exp. Bot. 59, 1569-1580. doi: 10.1093/jxb/ern084
Portis, A.R. (2003) Rubisco activase - Rubisco’s catalytic chaperone. Photosynth. Res. 75, 11-27. doi:10.1023/A:1022458108678
Salvucci, M.E., Portis, A.R., Ogren, W.L. (1985) A soluble chloroplast protein catalyzes ribulosebisphosphate carboxylase/oxygenase activation in vivo. Photosynth. Res. 7, 193-201. doi: 10.1007/BF00037012
Sulpice, R., Tschoep, H., von Korff, M., Büssis, D., Usadel, B., Höhne, M., Witucka-Wall, H., Altmann, T., Stitt, M., Gibon, Y. (2007) Description and applications of a rapid and sensitive non-radioactive microplate-based assay for maximum and initial activity of D-ribulose-1,5-bisphosphate carboxylase/oxygenase. Plant Cell Environ. 30, 1163-1175. doi:10.1111/j.1365-3040.2007.01679.x
Tsai, Y.C., Lapina, M.C., Bhushan, S., Mueller-Cajar, O. (2015) Identification and characterization of multiple rubisco activases in chemoautotrophic bacteria. Nat. Commun. 6, 1-10. doi: 10.1038/ncomms9883
Vitlin Gruber, A. and Feiz, L. (2018) Rubisco assembly in the chloroplast. Front. Mol. Biosci. 5, 24. doi: 10.3389/fmolb.2018.00024