Seasonal influenza virus vaccine effectiveness through recombinant neuraminidase on VLPs
JOINT-SUPERVISORS: Reingard GRABHERR and Alois JUNGBAUER
Background.
Trivalent and quadrivalent inactivated influenza virus vaccines (TIV, QIV) induce narrow, strain specific immune responses that target the immunodominant head domain of the viral hemagglutinin (HA). The influenza virus neuraminidase (NA) is also an excellent immune target as the NA harbors conserved epitopes (1, 2). Antibodies against the NA correlate with protection in humans (3, 4) and reduce viral shedding in infected individuals (5) and guinea pigs (6).
However, current vaccines are not efficient at inducing an immune response to the influenza virus NA (7, 8). The reasonsfor the lack of NA immunogenicity in TIV and QIV are not completely understood, but three major factors may contribute: (i) Low/non-standardized amounts of NA in the vaccine (7), (ii) NA in TIV and QIV might not display conserved conformational epitopes correctly due to denaturation during the vaccine production process and, (iii) NA is immune-subdominant to HA, especially when attached physically to HA (e.g. via the hydrophobic HA and NA transmembrane domains) (9, 10). A potential solution to this problem is to supplement the current TIV or QIV (or the recombinant influenza vaccine – RIV4) with correctly folded, stable, tetrameric recombinant NA protein (11), or alternatively with bionanoparticles that display tetrameric NA on an outer membrane.
Functional vaccines have already been produced on the basis of virus like particles (VLPs), e.g. formed by the assembly of HIV-1 capsid protein Gag (12). For the production of tetrameric soluble NA there already exists a baculovirus based construct, that has shown to be effective in mice. This construct has been developed by our collaborating partner Prof. Florian Krammer at the Icahn School of Medicine at Mount Sinai, NY, USA. Vaccination with this construct in the mouse model confers complete protection against a stringent 10x mLD50 challenge (unpublished data, personal communication). However, the construct is non-optimized for expression and is still purified using a hexahistidine tag. What is needed to fully develop this antigen for human use is a high yielding expression construct as well as development of an optimal upstream and downstream process (for the antigen without the tag). For the production of Gag based virus like particles, we have suitable baculovirus constructs, some of which show improved production of secreted proteins by co-expression of protein-disulfide isomerase. Since NA contains a high number of disulfide bonds, we will include this vector backbone in our test series. Naturally, NA is incorporated into the cellular membrane, therefore, budded virions will display the correctly folded NA just as it is displayed on the cellular surface.
The production processes for both, the soluble NA and the NA-VLPs will be developed in a novel Trichoplusia ni derived cell line (Tnms42) which has recently been characterized and confirmed to be free of any alphanoda virus contamination (in contrast to the commonly used High Five cell line) (13, 14). Especially for the production of pharmaceutical proteins that go into clinical studies, this cell line is favorable.
In terms of purification we face two challenges: (i) soluble NA has to be purified without containing an affinity tag and maintain its enzymatic activity and (ii) in the case of bionanoparticles we face the obstacle that extracellular vesicles are produced in parallel, that have identical surface properties and size as the VLPs. Separation of these two types of particles however, is essential for producing a vaccine suitable for clinical applications. While VLPs are basically an empty shell surrounded by the cell membrane, exosomes may contain proteins, RNA and/or DNA that often serve cell-to-cell communication, and thus is an unwanted feature in a vaccine formulation. Therefore, purification methods must be established to provide pure soluble NA as well as pure fractions of bionanoparticles.
After characterization and quality control, both vaccine candidates will be tested in mouse studies, where antibody titers will be compared as well as the induction of neutralizing antibodies and protection from challenge. This work will be done during a research stay (3-6 months) of the PhD candidate in the laboratory of Prof. Krammer.
Aims and methods.
The overall goal is to combine an optimal expression construct with a high yield production process and efficient purification methods that provide adequate material for mouse studies, in order to answer the question whether VLPs displaying NA are more suitable as vaccines against influenza virus as compared to soluble NA. We hypothesize that by presenting whole NA in its native form and concentrated on the surface of bionanoparticles we can increase the specific immune response in vivo. The advantages thereof are lower vaccine dosage and lower production costs maintaining the same protective effect.
The baculovirus insect cell system is highly suitable for the production of complex glycoproteins as well as for the production of VLPs. For the purpose of NA expression, baculovirus vectors will be optimized accordingly in terms of codon usage, secretion leader signal (e.g. gp64 versus mellitin leader) and co-expression of chaperons (protein disulfide isomerase). Three different NAs will be chosen as models, N1, N2 and NA from influenza B virus. Yield and quality of NA (enzymatic activity) will be the relevant criteria. Basically, two different expression platforms will be established (Figure 1), one of which produces complete NA on the surface of HIV-1 Gag based virus like particles, the other one will provide soluble tetrameric NA.
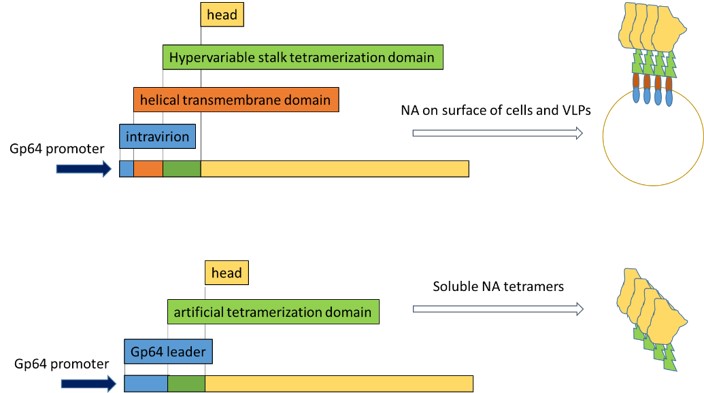
Fig. 1.In case of expressing the whole NA gene including the intravirion domain, the helical transmembrane domain, the hypervariable stalk domain and the antigenic head domain, native NA tetramers will be displayed on the surface of budded VLPs. When the helical transmembrane is being deleted and replaced by the gp64 leader signal and an artificial tetramerization site, NA will be secreted in a soluble form.
Further, a production process will be established in the insect cell line Tnms42, which already has been used for the generation of various VLPs in our hands. Shake flasks as well as a continuous production scheme have previously been used for production. Yield and enzymatic activity will be monitored during the process.
The soluble protein will be purified by a combination of mixed mode chromatography for capture using a positively charged resin followed by an anion-change chromatography with a linear pH gradient and a preparative size exclusion chromatography using Superose 6 to remove aberrant aggregated forms. The final purified material will be further concentrated by ultra/diafiltration. The VLPs will be purified by a method which has been previously developed in part at BIOTOP (15, 16). In brief, the culture supernatant is clarified by filtration, treated by Benzonase and then passed over a preparative random-access column such as Capto core. Further purification is achieved by Heparin affinity chromatography and anion-exchange chromatography with polymer grafted chromatography media. The finally purified material will be concentrated by ultra diafiltration. As in-process method HPLC SEC with multi angle light scattering will be used (17). For further biophysical characterization, field flow fractionation connected with multi angle light scattering and TEM will be used. Endotoxin level will be tested for those fractions, which will be subjected to animal testing. Testing for purity will be done by routine methods such HCP-ELISA, host cell DNA by qPCR and SDS-Page with Western blot analyses. Quantification of VLPs will be done by ELISA and Nanocyte, quantifying the number of NA molecules per VLP can be done by biolayer interferometry based techniques (18) in collaboration with Karola Vorauer-Uhl. Pre-clinical testing of the expressed antigens will be carried out in a mouse model (to be conducted in form of a research stay in the Krammer laboratory at the Icahn School of Medicine at Mount Sinai, New York).
Overall, the project offers to be involved and responsible for construction of genetic tools, setting up an insect cell culture expression process, develop and optimize new purification methods for soluble protein as well as for bionanoparticles as well as testing the in vivo efficacy of the final products (Figure 2). Thus, the PhD training includes various complementing disciplines, representing the whole production chain of a novel vaccine candidate (from design to challenge studies). On top of the educational value, the suggested platform is a highly promising and novel strategy for making modern universal influenza vaccines.
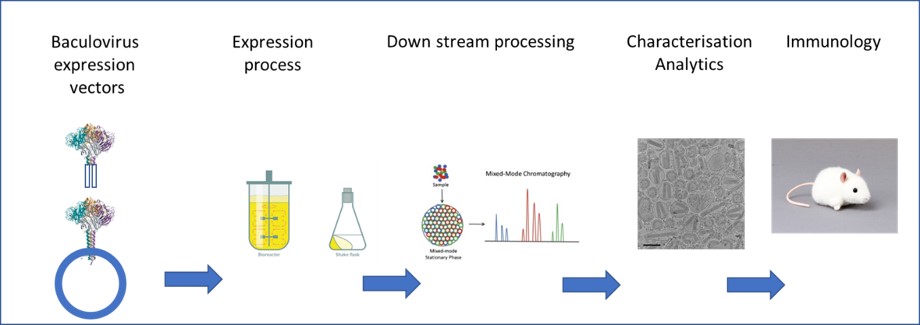
Fig. 2. work flow chart: genetic engineering and vector design for process development, followed by purification and analytics to end up with material for preclinical studies.
1.Y. Q. Chen et al., Influenza Infection in Humans Induces Broadly Cross‐Reactive and
Protective Neuraminidase‐Reactive Antibodies. Cell 173, 417‐429.e410 (2018).
2. T. J. Wohlbold et al., Broadly protective murine monoclonal antibodies against influenza B
virus target highly conserved neuraminidase epitopes. Nat Microbiol 2, 1415‐1424 (2017).
3. R. B. Couch et al., Antibody correlates and predictors of immunity to naturally occurring
influenza in humans and the importance of antibody to the neuraminidase. J Infect Dis 207,
974‐981 (2013).
4. A. S. Monto et al., Antibody to Influenza Virus Neuraminidase: An Independent Correlate of
Protection. J Infect Dis 212, 1191‐1199 (2015).
5. H. E. Maier et al., Pre‐existing anti‐neuraminidase antibodies are associated with shortened
duration of influenza A (H1N1)pdm virus shedding and illness in naturally infected adults.
Clin Infect Dis (2019).
6. M. McMahon et al., Mucosal Immunity against Neuraminidase Prevents Influenza B Virus
Transmission in Guinea Pigs. MBio 10 (2019).
7. T. J. Wohlbold et al., Vaccination with Adjuvanted Recombinant Neuraminidase Induces
Broad Heterologous, but Not Heterosubtypic, Cross‐Protection against Influenza Virus
Infection in Mice. MBio 6 (2015).
8. F. Krammer et al., NAction! How Can Neuraminidase‐Based Immunity Contribute to Better
Influenza Virus Vaccines? MBio 9 (2018).
9. B. E. Johansson, T. M. Moran, E. D. Kilbourne, Antigen‐presenting B cells and helper T cells
cooperatively mediate intravirionic antigenic competition between influenza A virus surface
glycoproteins. Proc Natl Acad Sci U S A 84, 6869‐6873 (1987).
10. B. E. Johansson, E. D. Kilbourne, Dissociation of influenza virus hemagglutinin and
neuraminidase eliminates their intravirionic antigenic competition. J Virol 67, 5721‐5723
(1993).
11. F. Krammer, P. Palese, Advances in the development of influenza virus vaccines. Nat Rev
Drug Discov 14, 167‐182 (2015).
12. Nika, L., Cuadrado‐Castano, S., Arunkumar, G. A., Grünwald‐Gruber, C., McMahon, M.,
Koczka, K., Garcia‐Sastre, A., Krammer, F., Grabherr, R. An HER2‐displaying virus‐like particle
vaccine protects from challenge with mammary carcinoma cells in a mouse model. Vaccines
7, article number 41 (2019).
13. Granados, R., Blissard, G., Debbie, P., (2016) Cell Lines That Are Free of Viral Infection and
Methods for Their Production. Google Patents
14. Koczka, K., Peters, P., Ernst, W., Himmelbauer, H., Nika, L., Grabherr R. Comparative
transcriptome analysis of a Trichoplusia ni cell line revealsdistinct host responses to
intracellular and secreted protein products expressed by recombinant baculoviruses. J.
Biotechnology 270, 61‐69 (2018)
15. Reiter, K., Aguilar, P.P., Wetter, V., Steppert, P., Tover, A., Jungbauer, A.
Separation of virus‐like particles and extracellular vesicles by flow‐through and heparin
affinity chromatography (2019) J. Chromatography A, 1588, pp. 77‐84.
16. Pereira Aguilar, P., Schneider, T.A., Wetter, V., Maresch, D., Ling, W.L., Tover, A., Steppert,
P., Jungbauer, A. Polymer‐grafted chromatography media for the purification of enveloped
virus‐like particles, exemplified with HIV‐1 gag VLP Vaccine, published electronically (2019)
17. Pereira Aguilar, P., González‐Domínguez, I., Schneider, T.A., Gòdia, F., Cervera, L., Jungbauer,
A. At‐line multi‐angle light scattering detector for faster process development in enveloped
virus‐like particle purification J. Separation Science, 42, 2640‐2649 (2019).
18. Nika, L., Wallner, J., Palmberger, D., Koczka, K., Vorauer‐Uhl, K., Grabherr, R. Expression of
full‐length HER2 protein in Sf9 insect cells and its presentation on the surface of budded
virus‐like particles. Protein Expr. Purif. 136, 27‐38, (2017)