Effect of membrane organization on weak acid transport proteins
JOINT-SUPERVISORS: Karola Vorauer-Uhl and Michael Sauer
Background.
Life is stressful. This general rule is true from microorganisms to humans. However, nature developed many mechanisms for life to cope with stress.
The membrane, separating the (stressful) outside world from the precious life inside of the cell, plays a central role for keeping stress at bay. Membrane transport proteins are the central gateways controlling which substances enter the cell and which substances are expelled, while the membrane itself serves as strong barrier to exchange.
Changing environmental conditions, for example changing pH values and changing concentrations of weak acids, which have the undesired potential to pass the membranes uncontrolled, lead to a chemical as well as biophysical adaptation of the membrane – to keep unwanted molecules out, as far as possible. Yeast cells change their membrane composition for example upon exposure to acetic acid, impeding the undesired penetration of the acid (Guo, 2018; Lindahl, 2016).
Furthermore, it has been shown that changing environmental conditions promote a change in the setup of membrane proteins by transcriptional and translational modulations, assuring the export of molecules which entered but also to sustain the required import of molecules, which are needed (Siontorou, 2017). While changes in the physicochemical properties of membranes and the genetic setup of the array of transport proteins are increasingly characterized, the direct impact of the physicochemical membrane properties on the characteristics of the present transport proteins themselves is not understood yet.
We hypothesize that the membrane composition not only affects the permeability of the membrane but also the functioning of the transport proteins. In order to shed some light on this question the aim of this collaborative project is to characterize the impact of the membrane composition on selected acetic acid transport proteins of baker’s yeast cells.
The first examples will be the acetate permease Ady2 (Ato1) and its paralog Ato2. These proteins are involved in acetate transport and mutations are prone to increase or decrease sensitivity to acetic acid (Paiva, 2004; Pacheco, 2012; Zhang, 2017). Furthermore, it has been shown that pH affects Ato1p localization in patches in the cell membrane (Ricicová, 2007). So, changes in the biophysical membrane environment, caused by changing pH, modifies the localization of this protein. However, if also the activity is modulated remains elusive and shall be analyzed in this project.
However, the membrane composition of living yeast cells cannot be modified in all desired ways to allow a direct analysis. Furthermore, a plethora of transport proteins are expressed at any time – many of them still uncharacterized - thereby impeding the analysis of the function of individual proteins. This project focuses therefore on a protocol for in-vitro membrane protein expression into advanced artificial liposomes, which allows the analysis of individual transport proteins (Doktorova, 2018; Sato, 2019). Figure 1 shows the work flow of this protocol, which was recently established in collaboration of both labs proposing this project. The in-vitro translation and direct incorporation of the proteins into the membranes solves problems related to denaturation/renaturation cycles of other liposome based protocols. The method itself does not allow controlling the direction of the incorporation of the proteins, however this is not important for the assay development, based on equilibria. Therefore, this method allows analyzing the properties of transport proteins in various membrane contexts in detail.
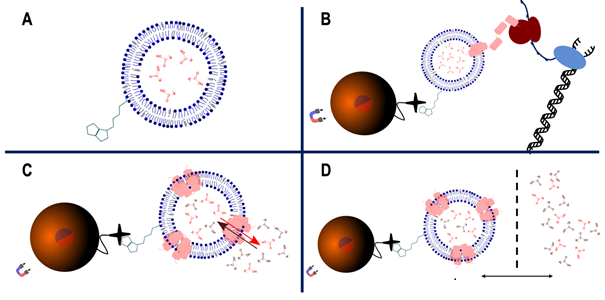
Fig. 1. A: artificial liposomes with controlled membrane composition are produced. They can be filled with substrates for the transport protein or detection systems, such as a pH indicator, fluorescent probes etc. Some lipids are biotinylated allowing the binding of the liposomes to magnetic beads. B: the transport protein is in-vitro translated and directly incorporated into the liposomes. This avoids all issues related to denaturation and renaturation of these proteins. C: the magnetic beads allow for an easy buffer exchange, allowing to change the environment from the translation mix to the buffer condition in which the transport phenomenon shall be analyzed. D: the liposomes can finally be isolated and the contents and supernatants individually analyzed.
Objectives.
Provision of liposomes made of yeast like membranes, comprising a choice of stress related membrane components. For the characterization, predominantly size/heterogeneity, surface charge, permeability, microdomain formation, protein-membrane partitioning, thermal phase transitions and membrane diffusion will be assessed. (Vorauer-Uhl)
In-vitro translation and incorporation of weak acid transport proteins into the liposomes. The first examples will be the acetate permease Ady2 (Ato1) and its paralog Ato2, others will be identified in the course of the project. (Sauer)
Analysis of the function of the transmembrane transport activity in dependence on the membrane composition. Functional assays rely on the one hand on analysis of the liposome content and the supernatant and on the other hand on the incorporation of readable test systems into the liposomes (such as pH indicators, fluorescent probes, enzymatic cascades). (Sauer)
When certain components have been identified, which modulate transport activity, the molecular details of the modulation shall be characterized – first of all determining the qualitative structure-activity-relation. In a second step a modelling approach may be chosen in collaboration with Prof. Oostenbrink. (Vorauer-Uhl)
Expected outcomes and impact.
This project explores the structure/function relationship of membrane proteins at molecular level which still remains largely elusive. As such the major question to be answered is, if the natural membrane remodeling impacts the respective transport proteins at all – if yes, the “how” shall be characterized in detail. The project outcome has therefore impact on our general understanding of transport proteins, cellular barriers and gateway functions.
More specifically, the project aims at molecular understanding of stress resistance in more detail and as such, addresses basic questions of our understanding of microorganisms in their natural habitats as well as industrial contexts. According to our hypothesis and the indications gained from observations in yeast cells, it seems plausible that complex cascades of membrane remodeling, favoring compounds such as ergosterol, which is forming lipid raft microdomains, attributes on the one hand a more tightly packed shielding membrane and on the other hand the incorporation of proteins such as Ady2. Our studies performed with varied artificial membranes, both membrane-related and protein effects can be elucidated in a distinct manner. Thus, our results are therefore of value for all cellular reactions, which involve membrane (re)organization.
Even more specifically, understanding of acetic acid stress has impact on how we preserve food, as particularly yeasts are typical spoilage organisms of pickled foods, because they can overcome acetic stress. At the same time the stress caused by acetic acid is one of the obstacles impeding efficient use of lignocellulosic hydrolysates with yeast cell factories. An understanding of this stress is therefore of use for industrial microbiology in context of biofuel production from second generation carbon sources.
Finally, compartmentalization by the plasma membrane is an integral feature of all forms of life. Deeper understanding of the functions is highly desirable. However, living organisms are immensely complex thus there is a need for simplified and controllable models for a deeper understanding of fundamental biological processes.
Collaborations
External:
Yvonne Perrie, University of Strathclyde, Strathclyde Institute of Pharmacy and Biomedical Sciences; Glasgow, UK; expert in Liposome Technology, Nanoparticle Synthesis and Microfluidic assisted Preparation
Margarida Casal, Center for Molecular and Environmental Biology, University of Minho, Portugal; expert in the analysis of weak acid transport
Internal:
Chris Oostenbrink, BOKU, Department of Material Sciences and Process Engineering, Expert in molecular modelling
Both BOKU, Department of Biotechnology, Muthgasse 18, A-1190 Vienna, Austria
Email: karola.vorauer-uhl@boku.ac.at, michael.sauer@boku.ac.at
Doktorova M, et.al. (2018) Preparation of asymmetric phospholipid vesicles for use as cell membrane models. Nat Protoc. 13(9):2086-2101. doi: 10.1038/s41596-018-0033-6.
Gentsch, M. et al. (2007) Mutations at different sites inmembers of the Gpr1/Fun34/YaaH protein family cause hypersensitivity to acetic acid in Saccharomyces cerevisiae as well as in Yarrowia lipolytica. FEMS Yeast Res. 7, 380–390. doi: 10.1111/j.1567-1364.2006.00191.x
Guo ZP, et al. (2018) Changes in lipid metabolism convey acid tolerance in Saccharomyces cerevisiae. Biotechnol Biofuels. 11:297. doi: 10.1186/s13068-018-1295-5.
Lindahl L, et al. (2016) Sphingolipids contribute to acetic acid resistance in Zygosaccharomyces bailii. Biotechnol Bioeng. 113(4):744-53. doi: 10.1002/bit.25845.
Paiva S, Devaux F, Barbosa S, Jacq C, Casal M. (2004) Ady2p is essential for the acetate permease activity in the yeast Saccharomyces cerevisiae. Yeast. 21(3):201-10. doi: 10.1002/yea.1056
Pacheco A, et al. (2012) Lactic acid production in Saccharomyces cerevisiae is modulated by expression of the monocarboxylate transporters Jen1 and Ady2. FEMS Yeast Res. 12(3):375-81. doi: 10.1111/j.1567-1364.2012. 00790.x
Ricicová M, et al. (2007) Association of putative ammonium exporters Ato with detergent-resistant compartments of plasma membrane during yeast colony development: pH affects Ato1p localisation in patches. Biochim Biophys Acta 1768(5):1170-8. doi: 10.1016/j.bbamem.2007.02.011
Siontorou CG, et al. (2017) Artificial Lipid Membranes: Past, Present, and Future. Membranes (Basel). 7(3). pii: E38. doi: 10.3390/membranes7030038.
Sato Y, Takinoue M. (2019) Creation of Artificial Cell-Like Structures Promoted by Microfluidics Technologies. Micromachines (Basel). 10(4). pii: E216. doi: 10.3390/mi10040216.
Zhang M, et al. (2017) Deletion of acetate transporter gene ADY2 improved tolerance of Saccharomyces cerevisiae against multiple stresses and enhanced ethanol production in the presence of acetic acid. Bioresour Technol. 245(Pt B):1461-1468. doi: 10.1016/j.biortech.2017.05.191.