Novel Lactate-Oxidizing Enzymes for Biosensor Applications
PRINCIPAL INVESTIGATOR: Dietmar HALTRICH
Background.
Biosensors based on lactate-oxidising enzymes are important for rapid clinical diagnostics. Monitoring the lactate concentration levels helps to assess patient health conditions but can also be applied in sports medicine, and shock or trauma analysis. The initial lactate level in blood is between 0.5 and 1.5 mmol/l but can rise to 25 mmol/l during intense exercising. Without sufficient clearance by the liver and kidneys, which usually takes only a few minutes, the accumulation of lactate leads to lactic acidosis. If disorders caused by lactic acidosis combine with one or more vital organ disorders, the patient develops severe sepsis. Recent studies have shown that early lactate clearance can improve treatment outcomes in severe sepsis and septic shock, which may lead to a decrease in sepsis-related mortality. Therefore, a patient's blood lactate level can serve as a signal for the severity of the disease and can also be used to improve the diagnosis and treatment of a wide range of diseases (Bakker et al. 1996; Kost 1993). In addition, rapid measurement of lactate concentration is also important for the food industry and could be of interest for the biotech industry (measure lactate levels in fermentation media).
Presently, the main enzyme used for lactate detection is L-lactate oxidase derived from Aerococcus viridans (AvLOx) (Kucherenko et al., 2019). Lactate oxidase is a member of the α-hydroxyacid-oxidase flavoenzyme family, and catalyses the flavin mononucleotide (FMN)-dependent oxidation of lactate while concomitantly oxygen is reduced to hydrogen peroxide (Rathee et al., 2016). Most of the biochemical knowledge on lactate oxidases was obtained for AvLOx, such as the elucidation of its structure (Umena et al., 2006), studies pertaining to structure/function relationships (Stoisser et al., 2015), or studies on its engineering (Minagawa et al., 2007). In contrast, LOx from other sources have rarely been studied to date. AvLOx it is not stable enough for long-term measurements and cannot be employed in a continuous lactate biosensor, and hence the identification of novel, better suited enzymes could be the starting point to construct novel lactate biosensors.
Aims and methods.
As preliminary work to this project we performed a BLAST search using the AvLOx amino acid sequence as a template, which resulted in retrieving more than 6000 homologous sequences. These were subsequently clustered by using sequence similarity networks. Based on this network we could pin down a LOx cluster containing approx. 500 sequences, mainly of Firmicutes and Proteobacteria origin, which was further analysed phylogenetically (Figure 1). Most of the clades in this phylogenetic tree are entirely composed of sequences that have never before been studied in any respect, so the sequence space of LOx is currently only poorly understood with respect to properties of its different members.
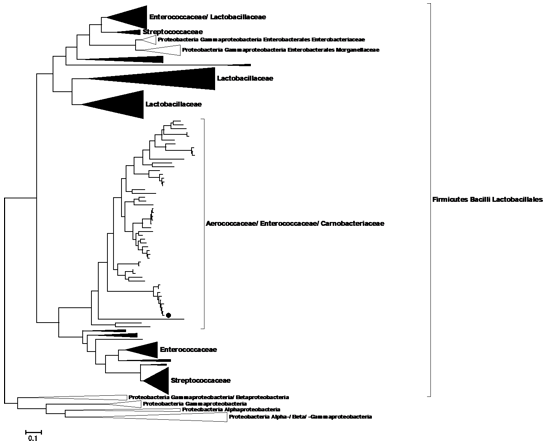
Figure 1. Phylogenetic tree of 500 sequences of the LOx Kluster calculated with FastTree. The black circle marks the position of AvLOx in the tree. White clades indicate Proteobacteria and filled black clades Firmicutes. With the exception of a Clade Enterobacterales, all Proteobacteria form an external group to the Firmicutes bacteria.
It is planned in this project to select a number of sequences from various clades of this LOx tree (~40-50) to cover the sequence space as wide as possible, to express the corresponding genes in E. coli, and to do a preliminary characterization of successfully expressed gene products (substrate specificity, stability). Selected enzymes (3–5) will then be studied in detail, again by (i) looking at the substrate specificity (are these true oxidases or will these enzymes also show significant dehydrogenase activity), (ii) kinetic properties (Michaelis-Menten steady-state kinetics as well as rapid kinetics), (iii) stability (DSC, inactivation kinetics during substrate turnover), (iv) their structure (crystallization and structure elucidation together with the core facility BmCA or with Lari Lehtiö, University of Oulu, Finland) and (v) their bioelectrochemical characteristics (redox potential, voltammetry; together with DirectSens). In a second part of the project, we will aim at tailoring the properties of LOx towards properties that are more suitable for biosensor applications, i.e., high specificity for lactate, suitable kinetic properties (high kcat) and high stability. To this end we will employ a novel high-throughput screening assay that is based on surface display of the oxidase on S. cerevisiae and detection of hydrogen peroxide formed by its reaction via a cell-bound roGFP2-Orp1 sensor (Herzog et al., 2020). This assay was developed as part of a previous BioToP project. For this second part of the project the work proposed will be (i) preparation of mutational libraries using random approaches; (ii) screening for increased stability and increased activity using FACS in combination with the roGFP2-Orp1-based screening assay; (iii) detailed measurement of thermodynamic and kinetic stability of variant LOx; (iv) steady-state kinetic analysis of variant LOx; (v) bio electrochemical characterization on suitable electrodes.
Overall, the scientific goal of the project is to get a better biochemical and enzymological understanding of lactate oxidases across their sequence space, and to understand structure/function relationships with respect to their kinetic properties and (operational) stability.
Collaboration within this thesis with include Roland Ludwig (bioelectrochemistry), Chris Oostenbrink (molecular modeling and dynamics), the core facility BmCA (DSC, FACS, crystallography), as well as Prof. Lari Lehtiö, Faculty of Biochemistry and Molecular Medicine, University of Oulu, Oulu, Finland (structure elucidation of LOx).
Bakker J, Gris P, Coffernils M, Kahn RJ, Vincent JL (1996) Serial blood lactate levels can predict the development of multiple organ failure following septic shock. Am J Surg 171(2):221-226, doi:10.1016/S0002-9610(97)89552-9
Herzog PL, Borghi E, Traxlmayr MW, Obinger C, Sikes HD, Peterbauer CK (2020) Developing a cell-bound detection system for the screening of oxidase activity using the fluorescent peroxide sensor roGFP2-Orp1. Protein Eng Des Sel 33. Gzaa019, doi:10.1093/protein/gzaa019
Kost GJ (1993) New whole blood analyzers and their impact on cardiac and critical care. Crit Rev Clin Lab Sci 30(2):153-202, doi:10.3109/10408369309084667
Kucherenko IS, Topolnikova YV, Soldatkin OO (2019) Advances in the biosensors for lactate and pyruvate detection for medical applications: A review. Trends Anal Chem 110:160-172, doi: 10.1016/j.trac.2018.11.004
Minagawa H, Yoshida Y, Kenmochi N, Furuichi M, Shimada J, Kaneko H (2007) Improving the thermal stability of lactate oxidase by directed evolution. Cell Mol Life Sci 64(1):77-81, doi:10.1007/s00018-006-6409-8
Rathee K, Dhull V, Dhull R, Singh S (2016) Biosensors based on electrochemical lactate detection: A comprehensive review. Biochem Biophys Rep 5:35-54, doi:10.1016/j.bbrep.2015.11.010
Stoisser T, Rainer D, Leitgeb S, Wilson DK, Nidetzky B (2015) The Ala95-to-Gly substitution in Aerococcus viridans l-lactate oxidase revisited - structural consequences at the catalytic site and effect on reactivity with O2 and other electron acceptors. FEBS J 282(3):562-578, doi:10.1111/febs.13162
Umena Y, Yorita K, Matsuoka T, Kita A, Fukui K, Morimoto Y (2006) The crystal structure of L-lactate oxidase from Aerococcus viridans at 2.1 Å resolution reveals the mechanism of strict substrate recognition. Biochem Biophys Res Comm 350 (2):249-256, doi: 10.1016/j.bbrc.2006.09.025